Most fish possess highly developed sense organs. Nearly all daylight fish have color vision that is at least as good as a human's (see vision in fish). Many fish also have chemoreceptors that are responsible for extraordinary senses of taste and smell. Although they have ears, many fish may not hear very well. Most fish have sensitive receptors that form the lateral line system, which detects gentle currents and vibrations, and senses the motion of nearby fish and prey.[1] Sharks can sense frequencies in the range of 25 to 50 Hz through their lateral line.[2]
Fish orient themselves using landmarks and may use mental maps based on multiple landmarks or symbols. Fish behavior in mazes reveals that they possess spatial memory and visual discrimination.[3]
Vision

Vision is an important sensory system for most species of fish. Fish eyes are similar to those of terrestrial vertebrates like birds and mammals, but have a more spherical lens. Their retinas generally have both rod cells and cone cells (for scotopic and photopic vision), and most species have colour vision. Some fish can see ultraviolet and some can see polarized light. Amongst jawless fish, the lamprey has well-developed eyes, while the hagfish has only primitive eyespots.[6] Fish vision shows adaptation to their visual environment, for example deep sea fishes have eyes suited to the dark environment.
Fish and other aquatic animals live in a different light environment than terrestrial species. Water absorbs light so that with increasing depth the amount of light available decreases quickly. The optic properties of water also lead to different wavelengths of light being absorbed to different degrees, for example light of long wavelengths (e.g. red, orange) is absorbed quite quickly compared to light of short wavelengths (blue, violet), though ultraviolet light (even shorter wavelength than blue) is absorbed quite quickly as well.[5] Besides these universal qualities of water, different bodies of water may absorb light of different wavelengths because of salts and other chemicals in the water.
Hearing
Hearing is an important sensory system for most species of fish. For example, in the family Batrachoididae, males use their swim bladders to make advertisement calls which females use to localize males. Hearing threshold and the ability to localize sound sources are reduced underwater, in which the speed of sound is faster than in air. Underwater hearing is by bone conduction, and localization of sound appears to depend on differences in amplitude detected by bone conduction.[7] As such, aquatic animals such as fish have a more specialized hearing apparatus that is effective underwater.[8]
Fish can sense sound through their lateral lines and their otoliths (ears). Some fishes, such as some species of carp and herring, hear through their swim bladders, which function rather like a hearing aid.[9]
Hearing is well-developed in carp, which have the Weberian organ, three specialized vertebral processes that transfer vibrations in the swim bladder to the inner ear.
Although it is hard to test sharks' hearing, they may have a sharp sense of hearing and can possibly hear prey many miles away.[10] A small opening on each side of their heads (not the spiracle) leads directly into the inner ear through a thin channel. The lateral line shows a similar arrangement, and is open to the environment via a series of openings called lateral line pores. This is a reminder of the common origin of these two vibration- and sound-detecting organs that are grouped together as the acoustico-lateralis system. In bony fish and tetrapods the external opening into the inner ear has been lost.
Current detection

The lateral line in fish and aquatic forms of amphibians is a detection system of water currents, consisting mostly of vortices. The lateral line is also sensitive to low-frequency vibrations. It is used primarily for navigation, hunting, and schooling. The mechanoreceptors are hair cells, the same mechanoreceptors for vestibular sense and hearing. Hair cells in fish are used to detect water movements around their bodies. These hair cells are embedded in a jelly-like protrusion called cupula. The hair cells therefore can not be seen and do not appear on the surface of skin. The receptors of the electrical sense are modified hair cells of the lateral line system.
Fish and some aquatic amphibians detect hydrodynamic stimuli via a lateral line. This system consists of an array of sensors called neuromasts along the length of the fish's body.[11] Neuromasts can be free-standing (superficial neuromasts) or within fluid-filled canals (canal neuromasts). The sensory cells within neuromasts are polarized hair cells contained within a gelatinous cupula.[12] The cupula, and the stereocilia which are the "hairs" of hair cells, are moved by a certain amount depending on the movement of the surrounding water. Afferent nerve fibers are excited or inhibited depending on whether the hair cells they arise from are deflected in the preferred or opposite direction. Lateral line neurons form somatotopic maps within the brain informing the fish of amplitude and direction of flow at different points along the body. These maps are located in the medial octavolateral nucleus (MON) of the medulla and in higher areas such as the torus semicircularis.[13]
Pressure detection
Pressure detection uses the organ of Weber, a system consisting of three appendages of vertebrae transferring changes in shape of the gas bladder to the middle ear. It can be used to regulate the buoyancy of the fish. Fish like the weather fish and other loaches are also known to respond to low pressure areas but they lack a swim bladder.
Chemoreception (smelling)

The aquatic equivalent to smelling in air is tasting in water. Many larger catfish have chemoreceptors across their entire bodies, which means they "taste" anything they touch and "smell" any chemicals in the water. "In catfish, gustation plays a primary role in the orientation and location of food".[14]
Salmon have a strong sense of smell. Speculation about whether odours provide homing cues, go back to the 19th century.[15] In 1951, Hasler hypothesised that, once in vicinity of the estuary or entrance to its birth river, salmon may use chemical cues which they can smell, and which are unique to their natal stream, as a mechanism to home onto the entrance of the stream.[16] In 1978, Hasler and his students convincingly showed that the way salmon locate their home rivers with such precision was indeed because they could recognise its characteristic smell. They further demonstrated that the smell of their river becomes imprinted in salmon when they transform into smolts, just before they migrate out to sea.[17][18][19] Homecoming salmon can also recognise characteristic smells in tributary streams as they move up the main river. They may also be sensitive to characteristic pheromones given off by juvenile conspecifics. There is evidence that they can "discriminate between two populations of their own species".[17][20]
Sharks have keen olfactory senses, located in the short duct (which is not fused, unlike bony fish) between the anterior and posterior nasal openings, with some species able to detect as little as one part per million of blood in seawater.[21] Sharks have the ability to determine the direction of a given scent based on the timing of scent detection in each nostril.[22] This is similar to the method mammals use to determine the direction of sound. They are more attracted to the chemicals found in the intestines of many species, and as a result often linger near or in sewage outfalls. Some species, such as nurse sharks, have external barbels that greatly increase their ability to sense prey.
The MHC genes are a group of genes present in many animals and important for the immune system; in general, offspring from parents with differing MHC genes have a stronger immune system. Fish are able to smell some aspect of the MHC genes of potential sex partners and prefer partners with MHC genes different from their own.[23]
Electroreception and magnetoreception

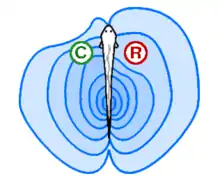
Electroreception is the ability to detect electric fields or currents. Some fish, such as catfish and sharks, have organs that detect weak electric potentials on the order of millivolts.[24] Other fish, like the South American electric fishes Gymnotiformes, can produce weak electric currents, which they use in navigation and social communication. In sharks, the ampullae of Lorenzini are electroreceptor organs. They number in the hundreds to thousands. Sharks use the ampullae of Lorenzini to detect the electromagnetic fields that all living things produce.[25] This helps sharks (particularly the hammerhead shark) find prey. The shark has the greatest electrical sensitivity of any animal. Sharks find prey hidden in sand by detecting the electric fields they produce. Ocean currents moving in the magnetic field of the Earth also generate electric fields that sharks can use for orientation and possibly navigation.[26] Among teleosts, the electric catfish uses electroreception to navigate through muddy waters. These fish make use of spectral changes and amplitude modulation to determine factors such shape, size, distance, velocity, and conductivity. The abilities of the electric fish to communicate and identify sex, age, and hierarchy within the species are also made possible through electric fields. EF gradients as low as 5nV/cm can be found in some saltwater weakly electric fish.[27] Several basal bony fishes, including the paddlefish (Polyodon spathula), possess electroreceptors. The paddlefish hunts plankton using thousands of tiny passive electroreceptors located on its extended snout, or rostrum. The paddlefish is able to detect electric fields that oscillate at 0.5–20 Hz, and large groups of plankton generate this type of signal.[28][29]
Magnetoreception is the ability to detect the direction one is facing based on the Earth's magnetic field. In 1988, researchers found iron, in the form of single domain magnetite, resides in the skulls of sockeye salmon. The quantities present are sufficient for magnetoception.[30]
Fish navigation
Salmon regularly migrate thousands of miles to and from their breeding grounds.[31]
Salmon spend their early life in rivers, and then swim out to sea where they live their adult lives and gain most of their body mass. After several years wandering huge distances in the ocean where they mature, most surviving salmons return to the same natal rivers to spawn. Usually they return with uncanny precision to the river where they were born: most of them swim up the rivers until they reach the very spawning ground that was their original birthplace.[17]
There are various theories about how this happens. One theory is that there are geomagnetic and chemical cues which the salmon use to guide them back to their birthplace. It is thought that, when they are in the ocean, they use magnetoception related to Earth's magnetic field to orient itself in the ocean and locate the general position of their natal river, and once close to the river, that they use their sense of smell to home in on the river entrance and even their natal spawning ground.[32]
Pain
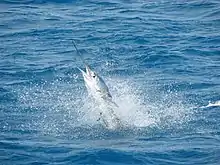
Experiments done by William Tavolga provide evidence that fish have pain and fear responses. For instance, in Tavolga's experiments, toadfish grunted when electrically shocked and over time they came to grunt at the mere sight of an electrode.[33]
In 2003, Scottish scientists at the University of Edinburgh and the Roslin Institute concluded that rainbow trout exhibit behaviors often associated with pain in other animals. Bee venom and acetic acid injected into the lips resulted in fish rocking their bodies and rubbing their lips along the sides and floors of their tanks, which the researchers concluded were attempts to relieve pain, similar to what mammals would do.[34][35][36] Neurons fired in a pattern resembling human neuronal patterns.[36]
Professor James D. Rose of the University of Wyoming claimed the study was flawed since it did not provide proof that fish possess "conscious awareness, particularly a kind of awareness that is meaningfully like ours".[37] Rose argues that since fish brains are so different from human brains, fish are probably not conscious in the manner humans are, so that reactions similar to human reactions to pain instead have other causes. Rose had published a study a year earlier arguing that fish cannot feel pain because their brains lack a neocortex.[38] However, animal behaviorist Temple Grandin argues that fish could still have consciousness without a neocortex because "different species can use different brain structures and systems to handle the same functions."[36]
Animal welfare advocates raise concerns about the possible suffering of fish caused by angling. Some countries, such as Germany have banned specific types of fishing, and the British RSPCA now prosecutes individuals who are cruel to fish.[39]
See also
References
- ↑ Orr, James (1999). Fish. Microsoft Encarta 99. ISBN 0-8114-2346-8.
- ↑ Popper, A.N.; C. Platt (1993). "Inner ear and lateral line". The Physiology of Fishes. CRC Press (1st ed).
- ↑ Journal of Undergraduate Life Sciences. "Appropriate maze methodology to study learning in fish" (PDF). Archived from the original (PDF) on 6 July 2011. Retrieved 28 May 2009.
- ↑ Land, M. F.; Nilsson, D. (2012). Animal Eyes. Oxford University Press. ISBN 9780199581146.
- 1 2 Helfman et al, 2009, pp. 84-87.
- ↑ N. A. Campbell and J. B. Reece (2005). Biology, Seventh Edition. Benjamin Cummings, San Francisco, California.
- ↑ Shupak A. Sharoni Z. Yanir Y. Keynan Y. Alfie Y. Halpern P. (January 2005). "Underwater Hearing and Sound Localization with and without an Air Interface". Otology & Neurotology. 26 (1): 127–130. doi:10.1097/00129492-200501000-00023. PMID 15699733. S2CID 26944504.
- ↑ Graham, Michael (1941). "Sense of Hearing in Fishes". Nature. 147 (3738): 779. Bibcode:1941Natur.147..779G. doi:10.1038/147779b0.
- ↑ B, WILLIAMS C. "Sense of Hearing in Fishes." Nature 147.3731 (n.d.): 543. Print.
- ↑ Martin, R. Aidan. "Hearing and Vibration Detection". Retrieved 1 June 2008.
- ↑ Bleckmann, H, and R Zelick. "Lateral line system of fish." Integrative Zoology 4 (2009): 13-25. doi:10.1111/j.1749-4877.2008.00131.x.
- ↑ Herring, Peter. The Biology of the Deep Ocean. New York: Oxford, 2002.
- ↑ Plachta D T T; Hanke W; Bleckmann H (2003). "A hydrodynamic topographic map in the midbrain of goldfish Carassius auratus". Journal of Experimental Biology. 206 (19): 3479–86. doi:10.1242/jeb.00582. PMID 12939378.
- ↑ Atema, Jelle (1980) "Chemical senses, chemical signals, and feeding behavior in fishes" pp. 57–101. In: Bardach, JE Fish behavior and its use in the capture and culture of fishes', The WorldFish Center, ISBN 978-971-02-0003-0.
- ↑ Trevanius 1822
- ↑ Hasler 1951
- 1 2 3 Moyle 2004, p.190
- ↑ Hasler 1978
- ↑ Dittman 1996
- ↑ Groot 1986
- ↑ Martin, R. Aidan. "Smell and Taste". ReefQuest Centre for Shark Research. Retrieved 21 August 2009.
- ↑ The Function of Bilateral Odor Arrival Time Differences in Olfactory Orientation of Sharks Archived 2012-03-08 at the Wayback Machine, Jayne M. Gardiner, Jelle Atema, Current Biology - 13 July 2010 (Vol. 20, Issue 13, pp. 1187-1191)
- ↑ Boehm T; Zufall F (February 2006). "MHC peptides and the sensory evaluation of genotype". Trends in Neurosciences. 29 (2): 100–7. doi:10.1016/j.tins.2005.11.006. PMID 16337283. S2CID 15621496.
- ↑ Albert, J.S., and W.G.R. Crampton. 2005. Electroreception and electrogenesis. pp. 431–472 in The Physiology of Fishes, 3rd Edition. D.H. Evans and J.B. Claiborne (eds.). CRC Press.
- ↑ Kalmijn AJ (1982). "Electric and magnetic field detection in elasmobranch fishes". Science. 218 (4575): 916–918. Bibcode:1982Sci...218..916K. doi:10.1126/science.7134985. PMID 7134985.
- ↑ Meyer CG; Holland KN; Papastamatiou YP (2005). "Sharks can detect changes in the geomagnetic field". Journal of the Royal Society, Interface. 2 (2): 129–30. doi:10.1098/rsif.2004.0021. PMC 1578252. PMID 16849172.
- ↑ Zimmerman, T., Smith, J., Paradiso, J., Allport, D., & Gershenfeld, N. (1995). Applying Electric Field Sensing to Human-Computer Interfaces. IEEE SIG .
- ↑ Russell DF; Wilkens LA; Moss F (November 1999). "Use of behavioural stochastic resonance by paddle fish for feeding". Nature. 402 (6759): 291–4. Bibcode:1999Natur.402..291R. doi:10.1038/46279. PMID 10580499. S2CID 4422490.
- ↑ Montgomery JC, Coombs S, Baker CF (2001) The mechanosensory lateral line system of the hypogean form of Astyanax fasciatus. Env Biol Fish 62:87–96
- ↑ Quinn 1988
- ↑ Dingle, Hugh; Drake, V. Alistair (2007). "What is migration?". BioScience. 57 (2): 113–121. doi:10.1641/B570206.
- ↑ Lohmann 2008
- ↑ Dunayer, Joan, "Fish: Sensitivity Beyond the Captor's Grasp," The Animals' Agenda, July/August 1991, pp. 12–18
- ↑ Vantressa Brown, "Fish Feel Pain, British Researchers Say," Agence France-Presse, 1 May 2003
- ↑ Kirby, Alex (30 April 2003). "Fish do feel pain, scientists say". BBC News. Retrieved 4 January 2010.
- 1 2 3 Grandin, Temple; Johnson, Catherine (2005). Animals in Translation. New York, New York: Scribner. pp. 183–184. ISBN 0-7432-4769-8.
- ↑ "Rose, J.D. 2003. A Critique of the paper: "Do fish have nociceptors: Evidence for the evolution of a vertebrate sensory system"" (PDF). Archived from the original (PDF) on 6 October 2009. Retrieved 21 May 2011.
- ↑ James D. Rose, Do Fish Feel Pain?, 2002. Retrieved 27 September 2007.
- ↑ Leake, J. (14 March 2004). "Anglers to Face RSPCA Check". The Sunday Times. Archived from the original on 23 September 2015. Retrieved 15 September 2015.
Further references
- Collin SP and Marshall NJ (Eds) (2003) Sensory Processing in Aquatic Environments Springer. ISBN 9780387955278.
- Green, W. W. and Zielinski B. S. (2013) "Chemoreception" In: D H Evans, J B Claiborne and S Currie (Eds) The Physiology of Fishes, 4th edition, pp. 345–373, CRC Press. ISBN 9781439880302.
- Popper AN and Fay RR (1993) "Sound detection and processing by fish: critical review and major research questions" (2 parts) Brain, behavior and evolution, 41 (1): 14–25. doi:10.1159/000338719 PDF part 1 PDF part 2
- Stevens, Martin (2013) Sensory Ecology, Behaviour, and Evolution Oxford University Press. ISBN 9780199601783.
- Webb JF, Fay RR and Popper AN (2008) Fish Bioacoustics Springer. ISBN 9780387730288.